Cavity Flow Studies using the HECToR Phase 2b System
S. Lawson, M. Woodgate, R. Steijl, and G. Barakos
CFD Laboratory, University of Liverpool
As part of the UK 2nd Applied Aerodynamics Consortium and the EPSRC project EP/C533380/1, the Multi-Block solver of Liverpool was used to analyse flows around Unmanned Combat Aerial Vehicle (UCAV) configurations that include bays for stores and sensors. This complex flow problem is usually studied using idealised configurations of flows inside rectangular cavities exposed to a stream of high speed flow. This problem is complex due to the strong interactions between the shear layer formed across the cavity and the acoustic waves radiated from the downstream cavity wall. This feedback mechanism is depicted in Figure 1 and can be seen with the flow snap-shots presented on Figure 2 where velocity dilatation is used to highlight the complexity of the flow-field inside the cavity. Due to the nature of this flow, at high Reynolds numbers and transonic conditions, Detached Eddy Simulation is a good technique for capturing the flow physics. The computations are demanding since these require long time-histories of the flow to be captured for the flow statistics to be extracted. Figure 3 shows comparisons against experimental data for the acoustics of the cavity obtained using the HECToR system. Computations of the idealised cavity typically require CFD grids of about 10 million cells but this size increases with the Reynolds number and the complexity of the flow geometry.
Figure 3 presents the flow field under a UCAV with a cavity exposed and Figure 4 shows comparisons between the CFD predictions and experiments for the sound pressure level inside the cavity. The overall good agreement between experiments and CFD can be further improved by increasing the size of the CFD model, especially as the complexity of the flow and UCAV geometry increases.
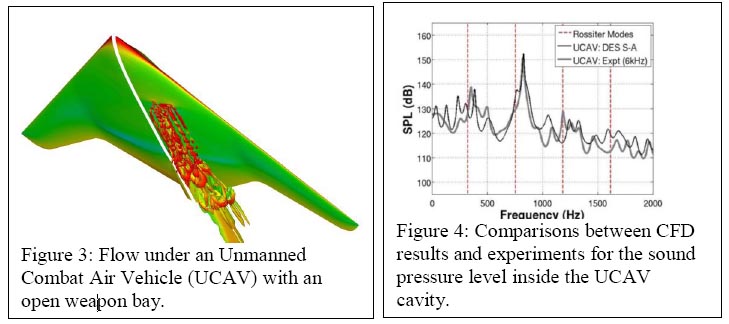
Larger CFD meshes have to be used for flows around complete unmanned aerial vehicles and this created the requirement for good code scalability on relatively large number of CPU cores. Phase 2B of HECToR provided the opportunity for testing the scalability of the Liverpool CFD solver on HECToR.
A DES grid of about 100 million points was constructed along with a scaled-up version of 1.1 billion points. Careful development of the parallel CFD methods was necessary to avoid memory bottlenecks and overlap as much as possible computation and inter-core communication. The solver was checked for performance using the HECToR system and the obtained speedup curves can be seen in Figure 5. Linear speedup is maintained for up to 8K cores using the 100 million points mesh and the performance on up to 24K deviated by just 12% from the ideal.
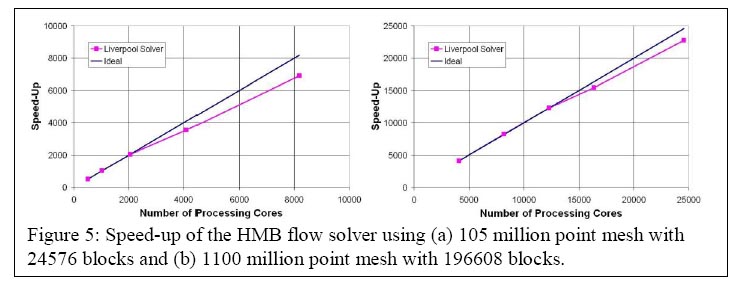
Even better results were obtained for the larger 1.1 billion point mesh. For this case, the deviation from the speedup was less than 6% on up to 24K cores. This result highlights the potential of the HECToR facility for large-scale studies into the physics of complex turbulent flows for configurations of practical importance. Further details about this research on cavity flows and UCAVs can be found in references [1-5].
Acknowledgements:
The financial support of EPSRC through grant EP/C533380/1 is gratefully acknowledged. The authors would like to thank Trevor Birch of DSTL for the experimental data and the UK 2nd Applied Aerodynamics Consortium for the use of HECToR.
References:
- S.J. Lawson and G.N. Barakos, CFD Analyses of Flow over Weapon-Bay Geometries, Journal of Aircraft, Accepted for Publication, 2010.
- S.J. Lawson and G.N. Barakos, Evaluation of DES for Weapons Bays in UCAVs, Aerospace Science and Technology, In Press, 2010, doi:10.1016/j.ast.2010.04.006.
- S.J. Lawson, G.N. Barakos and A. Simpson, Understanding Cavity Flows using Proper Orthogonal Decomposition and Signal Processing, Journal of Algorithms and Computational Technology, 4(1):47–69, March, 2010.
- G.N. Barakos, S.J. Lawson, R. Steijl and P. Nayyar, Numerical Simulations of High–Speed Turbulent Cavity Flows, Flow, Turbulence and Combustion, 83(4): 569–585, December, 2009, doi:10.1007/s10494-009-9207-1.
- S.J. Lawson and G.N. Barakos, Assessment of Passive Flow Control Devices for Transonic Cavity Flows Using Detached--Eddy Simulation, Journal of Aircraft, 46(3):1009–1029, May–June, 2009, doi:10.2514/1.39894.